A sustainable storage solution for the Science Museum Group
Article DOI: https://dx.doi.org/10.15180/150405
Abstract
Museums in recent years have sought ways to reduce the environmental impact of their operations. One approach has been to look at ways to cut back on the energy required to stabilise storage conditions, particularly relative humidity, through passive moisture control rather than mechanical systems of heating and air conditioning. To this end the Science Museum Group employed hemp in the form of hemp-lime concrete, to construct a new storage facility for its collections, drawing on research into the buffering ability of hygroscopic natural building materials. The objective was to reduce energy use, to decrease reliance on mechanical systems and to produce very stable levels of relative humidity, in order to ensure the preservation of significant heritage collections. Although a prototype, to date, this building has performed as anticipated despite some initial construction snags and mechanical system malfunctions. The results encourage further investigation into hygroscopic construction materials to design even more energy-saving ways of providing stable storage conditions for museums.
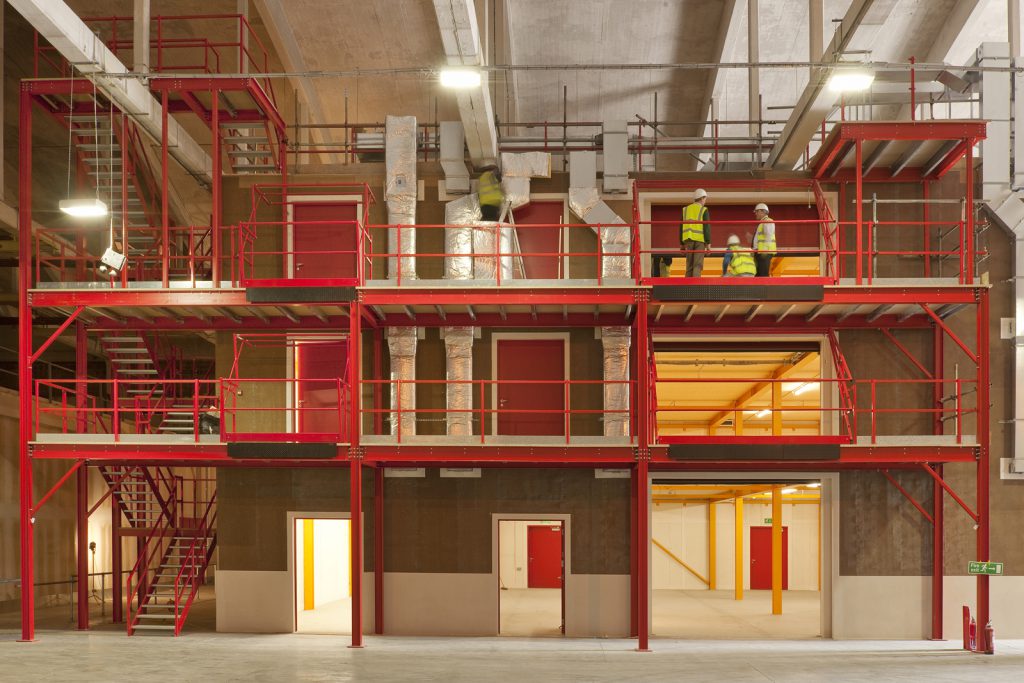
Keywords
hemp, hemp-lime concrete, hempcrete, hygroscopic building materials, museum storage, preventive conservation, relative humidity, sustainable
Introduction
https://dx.doi.org/10.15180/Heating and air-conditioning are probably the most significant consumers of fossil fuels by galleries and museums. We need to devise imaginative new solutions to resolve the dichotomy between long-term collections care and expensive environmental conditions.
In recent years museums have embraced sustainability, developing policies to reduce the carbon footprint of operations. Initiatives to lessen environmental impact by cutting back on energy and water use and increasing recycling and reuse have been introduced into day-to-day procedures. Larger scale schemes include sourcing materials and products with the least environmental impact (Moore, 2007) and encouraging contractors and suppliers to develop environmentally preferable goods and services (British Museum, 2007). Government directives to meet carbon emission reduction targets by improving overall building energy performance have resulted in minimum energy performance requirements for mechanical and engineering systems, such as heating and cooling, in new and in existing buildings (EU Directive, 2002). These are all worthwhile endeavours to enable museums to ‘go green’ and achieve environmental, economic and ethical benefits. But this paper argues that museums could go even further in conserving energy and reducing carbon emissions while actually improving their function as protective structures for cultural material. Renewable energy sources and natural construction materials could be used in the design of museum repositories which would not only substantially fulfil the directives for sustainability and reduction of carbon emissions but might also continuously maintain stable internal conditions which would assist in the preservation of heritage collections. This was the ambition that drove the Science Museum Group to pilot a novel form of sustainable storage.
Preventive conservation, also known as collections care, is one of the primary responsibilities of museums tasked with preserving material culture for posterity. The International Council of Museums – Committee for Conservation describes it as identifying and reducing potential risks and hazards to cultural and heritage objects while on exhibit and in storage by applying appropriate measures and actions for registration, storage, handling, packing and transportation, security, environmental management, emergency planning, staff and public awareness and legal compliance (ICOM-CC, 2008). By controlling light, temperature, relative humidity, pollution and pests, the need for interventive treatment can be forestalled. Both the levels and fluctuations of relative humidity (hereafter RH) must be moderated in order to mitigate damage not only to organic (animal and vegetal) materials but also to inorganics, particularly metals such as iron and copper alloys. The parameters recommended for stability in museum display and storage conditions, in particular those of temperature and RH have themselves recently been revised to a wider range than previously advised as a response to the need for sustainability (IIC-ICOM-CC, 2014). Proposed guidance to architects and engineers is for low energy and passive solutions to maintain the preservation needs of the collections instead of close control of prescribed levels through mechanical and engineering systems (NMDC, 2008b). Early research in domestic buildings suggests that stability of temperature and RH could be considerably enhanced by exploiting the buffering abilities of high thermal mass, thermal inertia and moisture absorption capability of building material and design (Osanyintola and Simonson, 2006); so far there has been limited investigation of the potential benefits to museums.
Building materials such as steel, concrete, brick, mineral wool insulation, glass, gypsum board and plastic paint are commonly used in the construction of museum buildings and storage repositories. However, while concrete, brick and insulation provide thermal capacity, none of them have any significant moisture transfer capability (Padfield and Larsen, 2004). A few low energy museum storage buildings have been constructed using such conventional materials but passive moisture buffering of the internal environment has been achieved more by the contents of the stores, chiefly paper, rather than by the hygroscopic or moisture absorbing ability of absorbent walls, ceilings and floors (Padfield et al, 2007).
There do exist more porous construction materials which have greater moisture absorbing capability than concrete, brick and gypsum. Among them are bio-based materials such as straw, flax and hemp, which also have excellent sustainable credentials; they are natural (renewable) resources, require less energy to produce and manufacture (low embodied energy), are recyclable; and lock up carbon dioxide (CO2) both during the growing phase and for the lifetime of a building (Lawrence, 2014). They have significant hygroscopic capability due to their porous structures and can buffer fluctuations of RH by releasing moisture as well as storing it, depending on ambient conditions. With the addition of low energy or passive systems of ventilation to and from external air, porous natural materials could potentially control RH to set points. The use of these materials for collections storage is, however, experimental, and in using them to create a new storage facility, the Science Museum Group needed not only to be aware of existing research, but undertake significant testing and monitoring of the new building. This work forms part of a PhD project by the author in conjunction with the University of Bath and is described in this article. The paper reports on the effectiveness of a natural porous building material, used in conjunction with a small air handling system, to provide the stable conditions and very low energy consumption required for a collections storage building.
The storage situation prior to the new building
https://dx.doi.org/10.15180/150405/002The Science Museum Group is devoted to the history and contemporary practice of science, medicine, technology, industry and media. With an unrivalled collection of historical and cultural material, it is considered to be the most significant group of museums of science and innovation worldwide (Science Museum, 2015). The Group consists of: the Science Museum (London), the Museum of Science and Industry (Manchester), the National Railway Museum (York), the National Media Museum (Bradford) and the National Railway Museum (Shildon). Its collection includes fine art, archival, photographic and library materials as well as thousands of objects both large and small, ranging for example from airframes and locomotives to surgical scalpels and needles.
As in many sizable museums, more than ninety per cent of the group’s collection is in storage rather than on display, despite having five public locations and a dynamic loans programme. Not all objects were collected to be displayed but were acquired for reference and research. Some objects remain in storage as they may be too large, too fragile or too hazardous to go on public display but are retained because of their cultural, historic or technical value.
Previously, each museum in the group had its own storage facilities both at the museum site and in external warehouses. But to reduce costs to the estate, by 2015 all northern external stores were closed and collections transported to the storage site at Wroughton, Wiltshire. Storage for the Science Museum Library & Archives had already been moved to Wroughton in 2007.
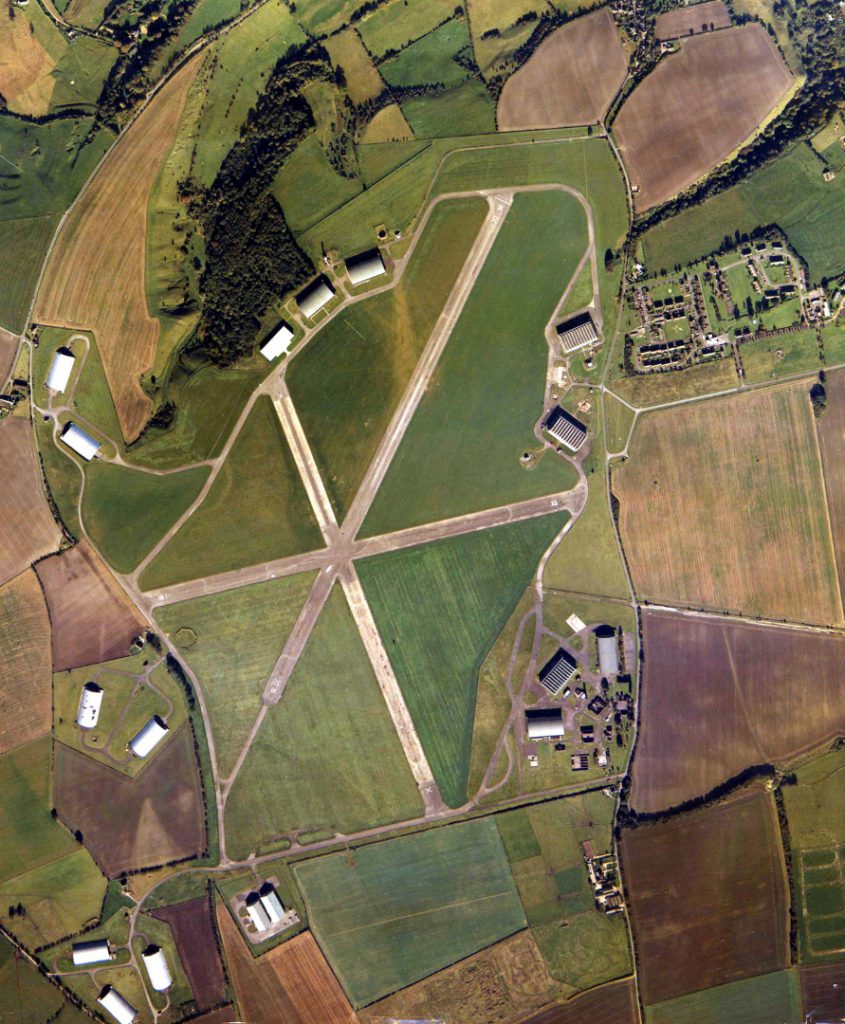
The Wroughton store is near Swindon on a former RAF airfield. The 545-acre site, purchased from the Ministry of Defence in 1979 for use as a large objects store, has ten Second World War hangars (with eight being used for collections storage) and one custom-built store (store A1, opened in November 1994). Former maintenance buildings have been converted for use by the Library & Archives, Conservation and Estates operations. At almost 200 metres above sea level the site is exposed to winds from all directions and is susceptible to frequent weather changes. Horizontal rain is a particular feature, resulting in moisture penetration of ageing building fabric.
The hangars, built in the late 1930s to three different designs all approximately 38,000 cubic metres in capacity, are not heated. All are uninsulated concrete and steel structures.
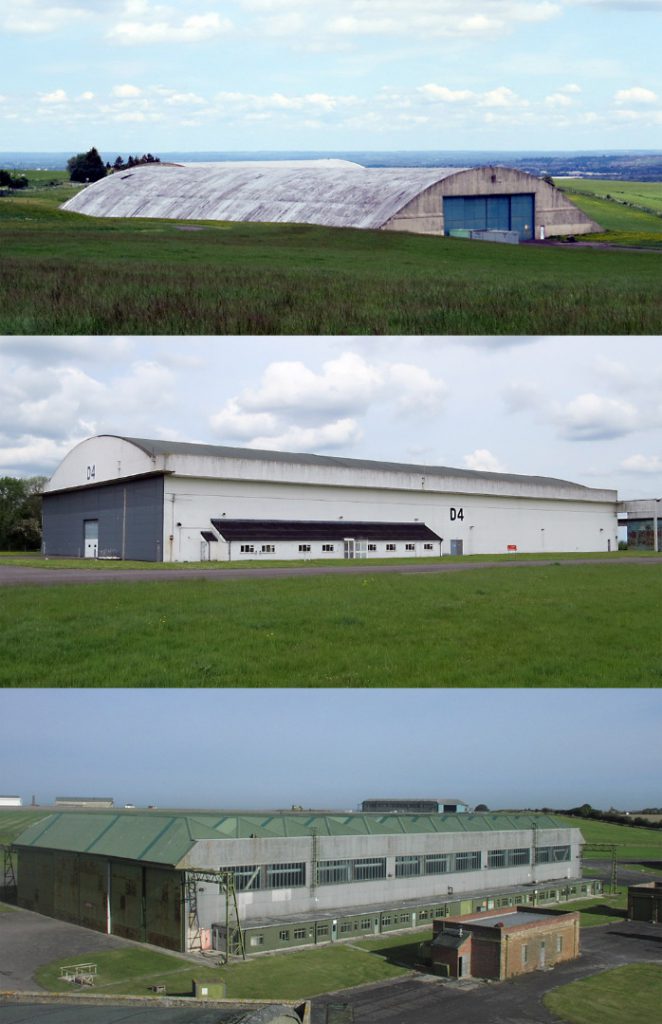
The hangers are in varying degrees of disrepair, some with very limited maintenance over the past 36 years, which has resulted in cracking concrete and rusting steel elements. Three have been renovated, one more fully than the others. However, with the site often being wet and windy, the environment in all the hangars is cold and damp, with temperatures rarely climbing above 13oC, and RH levels between 65–100 per cent, with internal fluctuations mirroring external conditions. Large industrial, agricultural and transport objects are stored either on the floor or on pallets in the hangars. Long-span pallet racking holds smaller or disassembled objects from the engineering technologies, medical, computing, media and science collections; these can be crated, covered with Tyvek or polythene, treated with surface coatings or unprotected, depending on object type and condition.
Store A1 is an insulated steel framework structure with concrete foundation and felt roof. It was built in 1992–93 in response to a strategic review of the Science Museum storage requirements. Designed with environmental controls provided by a building management system, with original specifications for temperature at 14±2oC and relative humidity of 55±5 per cent, the building failed to deliver the tight environmental parameters due mainly to the failure of the dehumidification system. Continuous low level heating by oil-fuelled boilers currently maintains the RH between an acceptable 45–65 per cent in the storerooms, but the boilers are ageing and require almost daily maintenance. Storage is on mobile and static racking, leaving no floor space for over-size objects, and there is almost no capacity left for additional objects in the racking. Clearly a new, economically and environmentally sustainable storage solution needed to be found.
Preventive conservation storage issues
https://dx.doi.org/10.15180/150405/003Storage issues for an extraordinary collection of science objects are complicated by both size and multiplicity of materials of many of the objects. Objects such as a 1918 Ammonia Refrigeration Compressor, a station gas meter and the first hovercraft, are large and robust and can withstand less than ideal environmental storage conditions. Many of the other 35,000 objects stored at Wroughton are made with materials which are vulnerable to light exposure, duration and wavelength; particulates; smoke and gaseous pollution; insect and rodent pests; and varying levels and fluctuations of temperature and RH. Many of the objects in storage at Wroughton are large and robust, such as the following example images:
Objects such as in the following images are not inconsiderable in size but are made from materials which can fade, are tempting to insect and other pests and can react disastrously to changes, especially in RH:
While it is true that greater damage and deterioration to the museum collection could come from fire, flood, accident, theft, vandalism and conflict, occurrences of these are far less likely due to site location and preventive measures.
While damage from light intensity and duration within the hangars is eliminated by excluding all light except during occupation, the levels of RH and temperature (hereafter T) within the hangars do not meet the museums’ own agreed recommendations for best practice collections care for robust objects (RH 35–65±20 per cent; T 16oC–24oC±3oC (Brain and Burden, 2011)). Through the annual assessment of storage spaces, the issues of incorrect T and RH and pest management have been highlighted by the conservation and collections care team. All issues are a result of the ageing structures which were never intended either to still be in use or to be used to store objects of cultural significance. Pests – birds, particularly jackdaws and pigeons, and rats, mice and rabbits – find their way into the hangars through holes at the top and bottom of the corroding hangar doors. Unless food or nesting material is present, little damage is done to the objects by rodents. However, bird excrement can damage surface finishes so preventive measures such as covering vulnerable objects with Tyvek sheeting are undertaken. Moths and mould flourish under favourable conditions of high RH and warm T but the hangars are rarely warm; however, outbreaks of both moth and mould have occurred, especially in road vehicles with fabric and leather interiors.
In fact, serendipitously, the cold temperatures could slow down the rate of deterioration in materials. Research has shown that organic material such as paper decays more slowly at temperatures under 12oC (Burge, 2014; Michalski, 2002; Wilson, 1995). It is the associated high RH levels in the hangars that cause most of the deterioration to the collection, whether objects are composed of organic or inorganic materials, or both. It is therefore the issue of controlling humidity that was the primary concern that any new storage facility needed to address.
Deterioration caused by relative humidity
https://dx.doi.org/10.15180/150405/004For each material, there is a level of environmental moisture content, related to the associated relative humidity, which is consistent with its physical, chemical or biological stability.
Relative Humidity is the ratio of current absolute humidity in the air relative to the maximum absolute humidity at a particular temperature. As warm air can hold more moisture than cold air, RH drops as temperature rises and conversely rises as temperature drops. At 100 per cent RH, the air is saturated and the moisture can condense into water droplets. Changes in RH can cause physical, chemical and biological damage to the materials out of which objects are made.
In materials derived from plant and animal matter (organic materials), which naturally contain water, moisture content adjusts to equilibrate with the RH of the environment, and there can be subsequent changes in dimension or physical characteristics which can result in physical damage. Damage from moisture gain can comprise swelling, cockling, warping, plasticising, leaching, dissolving, fading, staining, weathering, cracking and weakening. Conversely damage from moisture loss can result in shrinking, splitting, cracking, breaking, crumbling, delaminating, stiffening and weakening. The extent of damage will depend on the internal physical structure of a material, any external constrictions, the environmental RH values and rates of change, and overall time of exposure.
Chemical damage can also occur to both organic and inorganic materials at high humidity levels. Organic materials may lose strength, colour, shape and flexibility when chemical bonds are broken by the addition of a water molecule (hydrolysis). With low RH levels, salt contaminated ceramics, metals and stone may develop white crystals on their surface (efflorescence) or even disintegrate, and unstable glass may turn opaque (crizzle). In high RH levels salts from deteriorating glass can attract moisture, with droplets forming on the glass surface, and metals can corrode; a layer of dust will accelerate corrosion rates when the RH rises above 75 per cent. Biological deterioration is mostly caused by micro fungal growth (mould); some moulds will germinate around 65 per cent RH with a sufficiently warm temperature. Mould can grow on both organic and inorganic materials if there are suitable nutrients available.
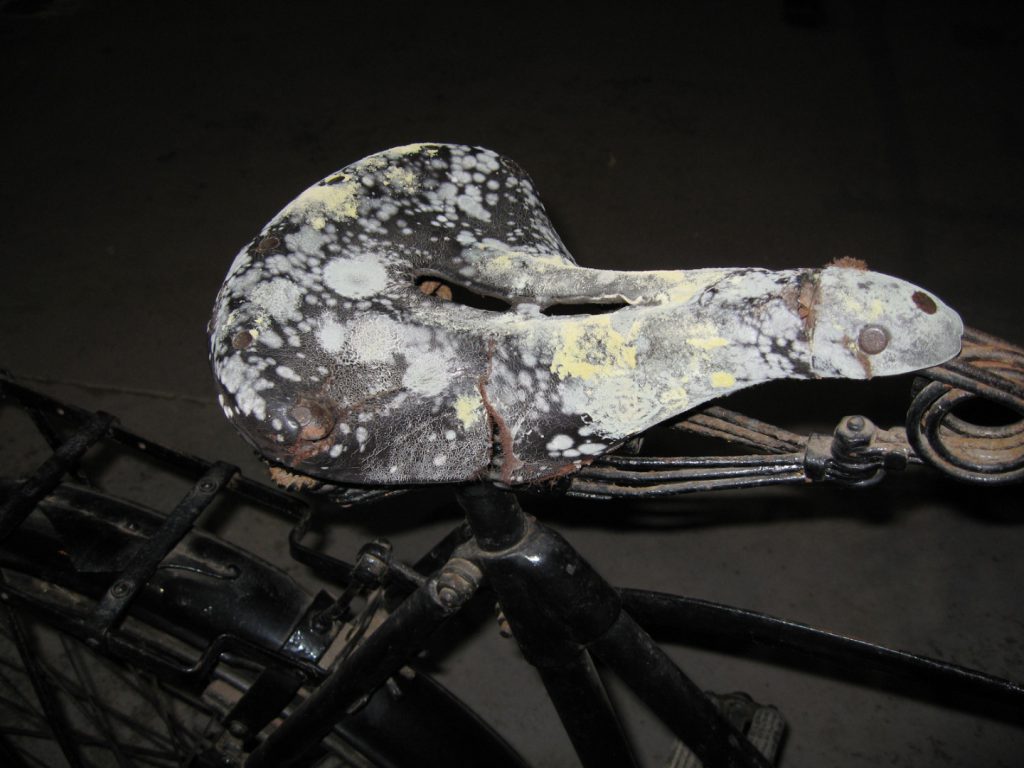
For mixed material museum collection preservation, the museum group RH guidelines agree with the wider guidelines, set out in the recent joint declaration from the International Institute for Conservation and the International Council of Museums – Conservation Committee, advocating a moderate range of 40–60 per cent ±5–10 per cent. While RH levels in the A1 store at Wroughton remain within the set points due to consistent low-level heating, RH in the hangars rarely drops to the highest end point. Even the most extensively renovated hangar has higher than acceptable levels of humidity for much of the year. Replacing the old hangars with suitable buildings for the storage of the group’s collection is the ultimate solution. Designing sustainable storage buildings to museum preventive conservation policy guidelines is the challenge.
The need for a new kind of museum store
https://dx.doi.org/10.15180/150405/005In 2010 an agreement to house the incipient Rail Industry National Archive as well as a need for environmentally controlled storage for paintings, ship models and horse-drawn carriages[1] prompted the decision to build a new store at Wroughton. The collections all required stable RH levels which could be allowed to drift moderately within the recommended set-points but the levels had to be provided by low energy consumption and reduced reliance on a mechanical and engineering system (Moore, 2013). The store would be constructed inside one of the renovated hangars (Hangar D2) which already held the archive store; planning permission would not be required and it was thought the hangar itself would provide an additional level of protection from the outside elements.
The design project team had members from various museum departments: Estates, Sustainable Development, Conservation & Collections Care and Curatorial (Archives). Input from Conservation and Sustainable Development led the project team to consider the use of a hygroscopic building material which would buffer RH fluctuations sufficiently to eliminate constant reliance on heating or dehumidification. Based on research from the University of Bath (Sutton et al, 2011) and results from newly constructed commercial warehouses (Bevan and Woolley, 2009; Betts, 2010), a bio-based construction concrete made from industrial hemp and lime binder was chosen, both for its buffering ability and its ecological credentials.
Hemp-lime concrete: what is it?
https://dx.doi.org/10.15180/150405/006Hemp-lime concrete or hempcrete is a mix of the chopped up woody stalk (shiv) of industrial hemp shiv (Cannabis sativa) with a lime-based binder and water.
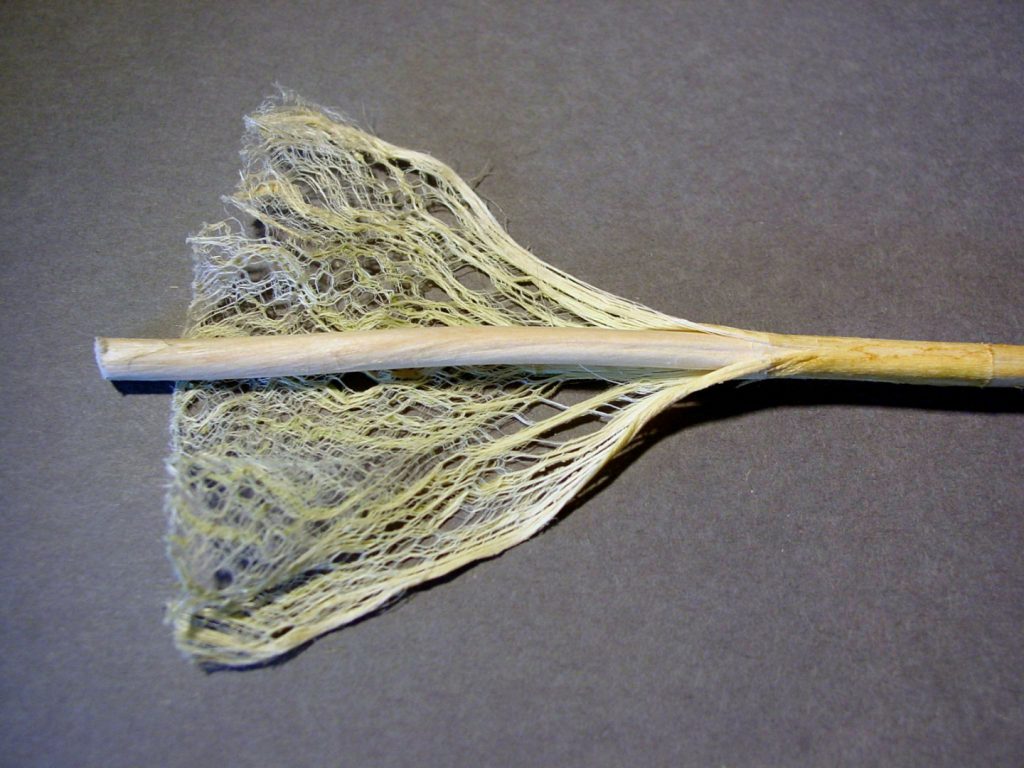
Industrial hemp is a fibrous plant similar to flax and jute. It is a fast-growing (three to four months) carbon sequestrating broad-leafed plant which requires minimal fertilisers, pesticides and herbicides and only moderate amounts of moisture. Hemp was first harvested by the Chinese over 8,500 years ago, then introduced to west Asia, Egypt and Europe between 2000 and 1000 BCE (Small and Marcus, 2002). Industrial hemp is grown for fibre and grain: fibres are used to produce textiles (the name ‘canvas’ is derived from cannabis), string and rope, and paper; grain is used for food, animal feed and oil. While of course it comes from the same family as the cannabis drug, breeding hemp over centuries for tall slender plants with long fibres has reduced the tetrahydrocannabinol (THC) content (the psychoactive ingredient) to less than 0.3 per cent, making the plant a poor source of drug material. China leads the world in the production of industrial hemp; it is grown in over thirty countries including the United Kingdom, where it must be grown under the Home Office Low THC Cannabis (Hemp) licence.
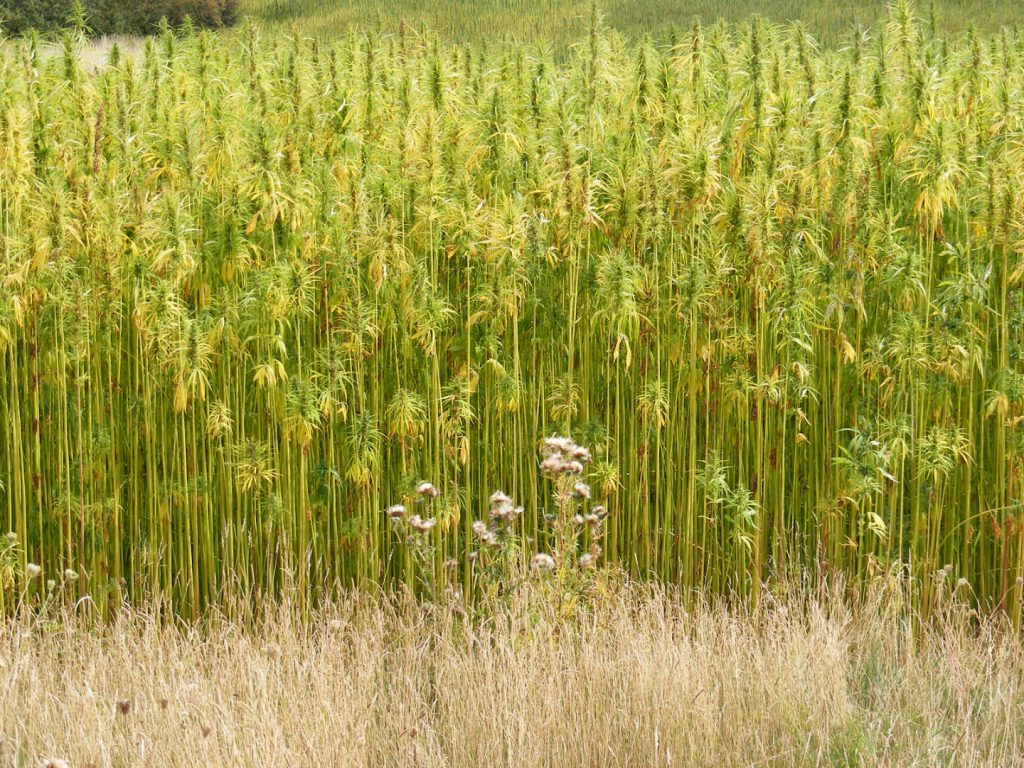
Once a crop is cut, the hemp stalks are left in the field until sufficiently rotted (retted) by rain and dew; then they are baled and stored indoors to allow drying to halt the retting process. The outer fibres are separated from the inner woody shiv using a decorticator, which also shreds the shiv into chips. The chips, originally considered to be a low-value waste product of the fibre industry, predominately marketed as animal bedding, have begun to be used as a bio-based construction material. By 2004, about four per cent of the hemp shiv produced in the EU was used in construction (Karus and Vogt, 2004).
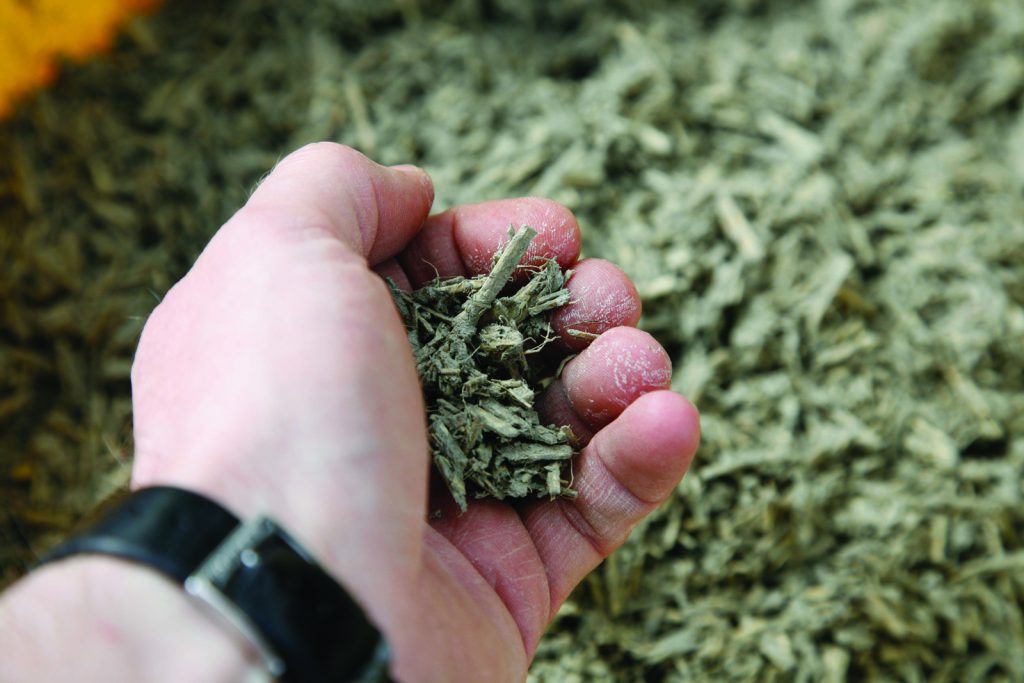
Hemp as a building material
https://dx.doi.org/10.15180/150405/007Hemp shiv has characteristics that make it particularly suitable as a building material. It is more resistant to biological decay than similar bio-based materials such as straw and its silica content makes it fire-retardant. Shiv is a very porous material, being approximately sixty per cent air by volume, which makes it very hygroscopic, attracting (adsorbing) moisture quickly and releasing it (desorbing) slowly. It is hydrophilic, absorbing water up to four times its weight. Thermal conductivity is also low due to the internal porosity, which retards the rate of heat transfer as air is less conductive (Arnaud and Gourlay, 2012).
However, the fibres require a binding material to produce a mortar. As hempcrete was initially used as a sympathetic repair material for historic oak framed buildings infilled with a mixture of straw and lime (wattle and daub) (Hirst et al, 2010) lime-based binders have been used in the mix in preference to Portland cement. Portland cement, which sets hard and relatively quickly and requires less skill to use, replaced the use of lime mortars from the middle of the nineteenth century (Carran et al, 2011). But, because cement’s hardness and density is incompatible and damaging when used with any ‘soft’ and porous building material such as traditional brick or porous sedimentary stone, lime mortar production has recently been revived for the conservation and restoration of heritage buildings and for use with other natural building materials (Labesse, 2005). Commercially available lime binders typically contain a varying proportion of natural hydraulic lime, hydrated or slaked lime, a hydraulic component such as Portland cement and/or other silica-containing materials such as fly ash, a residue of coal burning. Fly ash improves the workability of the mix due to its fine round grains and reduces the required water content (De Bruijn and Johansson, 2013).
Benefits of using lime
https://dx.doi.org/10.15180/150405/008Despite the potential environmental impact of limestone mining and lime production, there are many benefits to using lime. It is vapour permeable, which enhances control of RH and reduces the potential for mould growth and condensation; it has low thermal conductivity and can act as a massive thermal heat store; it is alkaline, inhibiting mould growth and insect activity; it is long-lasting; it is fire and water resistant; it can easily be recycled or absorbed back into the land without toxicity (Rhydwen, 2009). Hydrated lime mortar is known for being self-healing’; as it cures slowly through carbonation[2], it can adjust to early building movement. If appropriate wet-dry cycles are available, the calcium compounds can reprecipitate into cracks, then continue the carbonation process (Lubelli et al, 2011).
The advantages of using hemp-lime concrete
https://dx.doi.org/10.15180/150405/009The sustainable credentials of hemp-lime are substantial: renewable, recyclable, potentially sealing up enough carbon dioxide to produce a negative carbon footprint in construction (Lawrence et al, 2012), but the crucial benefit of using hempcrete for museum storage is its hygrothermal – heat and moisture – buffering behaviour. Its low thermal conductivity and good heat capacity provides excellent insulation. Its water vapour permeability, high moisture transfer and storage capacity moderate the humidity of the ambient environment and provide the crucial consistency of environment that objects need.
Hempcrete has a range of building uses from roof insulation (low density) to walls (medium density) and ground slabs (high density), produced by varying the proportions of binder, hemp and water. Different densities have a significant effect on the movement of heat and moisture through the material, with lower densities being more porous, which results in lower thermal conductivity and therefore better insulating performance (Barclay et al, 2014). There are good connections between the pores, which make it highly permeable and able to absorb moisture and sound. Hempcrete’s overall buffering effect on indoor RH has been shown to improve respiratory comfort, skin humidity and perceived indoor air quality (Collet et al, 2013). Moisture buffering also reduces condensation and mould growth, decreasing the need for ventilation and cutting energy costs (Colinart et al, 2013; Latif, 2014). Despite low density hempcrete requiring a load bearing structure, its benefits make it a very attractive material for constructing museum storage.
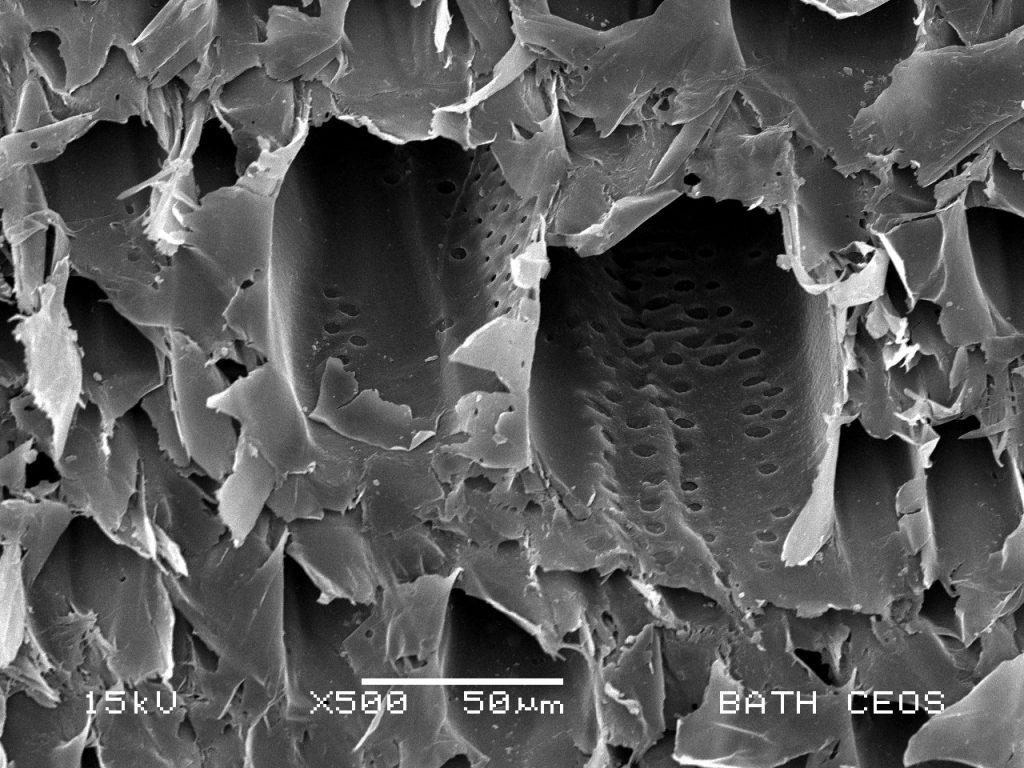
Hempcrete Museum Store design and construction
https://dx.doi.org/10.15180/150405/010Having selected the building material, the museum appointed contractors whose approach supported the intent to produce a low cost, low-energy and sustainable storage building. The building design was modelled with Integrated Environmental Solutions (IES) software using environmental data from the hangar interior and the site, and the known thermal efficiency and hygroscopic performance of hempcrete in order to produce a reduced air handling system. In fact, the software did not accurately simulate the hygroscopic behaviour of the hempcrete and a numerical procedure was therefore developed to adjust the parameters of simulated temperature and humidity to bring them closer to results from laboratory tests. This resulted in the design of a heating and ventilation system with no active control of humidity and no active cooling mechanism, but with expectations of meeting an RH level of 50±5 per cent most of the time (Jankovic, 2012) by relying on the passive buffering performance of the hempcrete walls and on seasonal ambient humidity levels.
A steel-framed three-storey, six-room repository, providing 980m2 of storage space, was planned, taking full advantage of the height within the hangar.
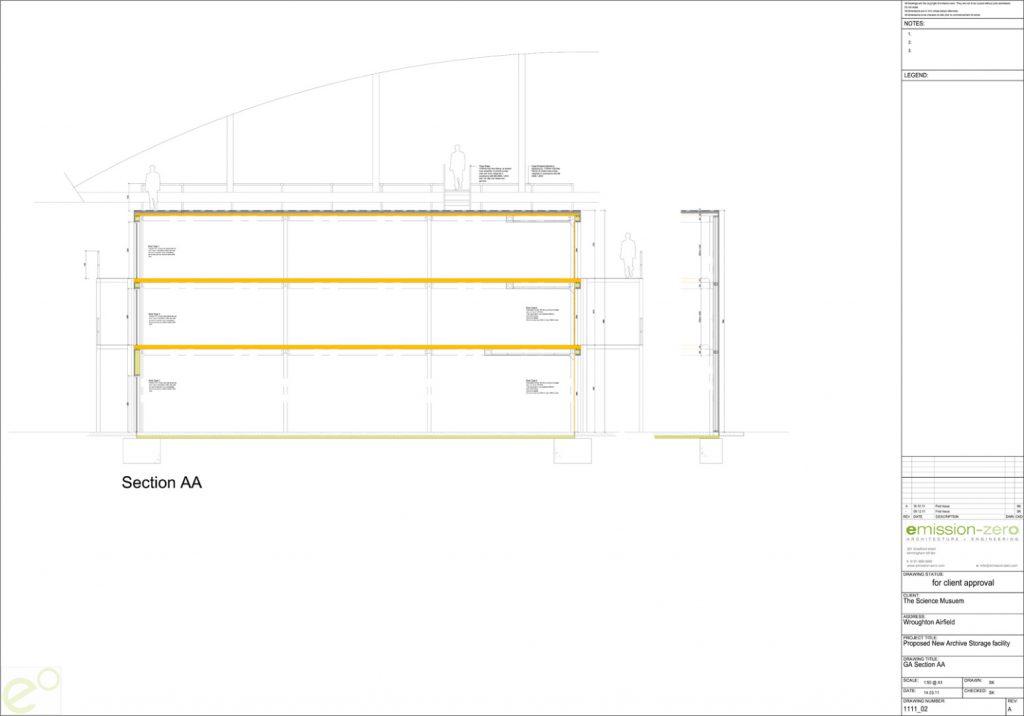
Ceiling height on each level was dictated by the needs of the collections and the rafters of the hangar; the ground level ceiling had to be high enough for the tallest of the horse-drawn carriages, which reduced the height of the other two levels to just under average ceiling heights (225 cm). Cross-laminated timbers, formed from spruce panels bonded together perpendicularly to one another, would give the floors and ceilings high structural strength, excellent dimensional stability and vapour permeability (Sutton et al, 2011) The panel sections would be inserted into the steel framework, speeding up construction time and eliminating site waste. Before the steel framework was erected, the original hangar floor had to be removed and a new floor poured with deeper foundations for the support columns as a high floor load had been specified for the archival storerooms.
Because the storage repository was being built inside a hangar which would mitigate wind chill and some temperature extremes, the focus was on thermal inertia and humidity control rather than temperature regulation. The contractor fitted a prefabricated cladding system, Hemclad®, coupled with Hemcrete® in timber framed panels, over the primary steel frame structure. While the cladding system normally had a thickness of 120mm and included a hemp fibre quilt for thermal insulation, the system was modified to eliminate the fibre quilt and increase panel thickness to 200mm to augment moisture and thermal buffering capacities.
Hemcrete® is a proprietary blend of lime-based binder and prepared hemp shiv, mixed with water to form a slurry for casting or spraying. Hemcrete® panels are factory produced, force-dried and available in bespoke sizes. The panels meet current British Standard fire resistance tests for building materials. Pre-cast panels are intended for year-round construction and where time on site is restricted. While hemp-lime mix can be produced wet on site and either cast inside a framework or sprayed against a lining board, as there was a tight deadline due to the storage needs of the collections, construction of the store had to take place over the winter months and pre-cast panels were the only construction choice.
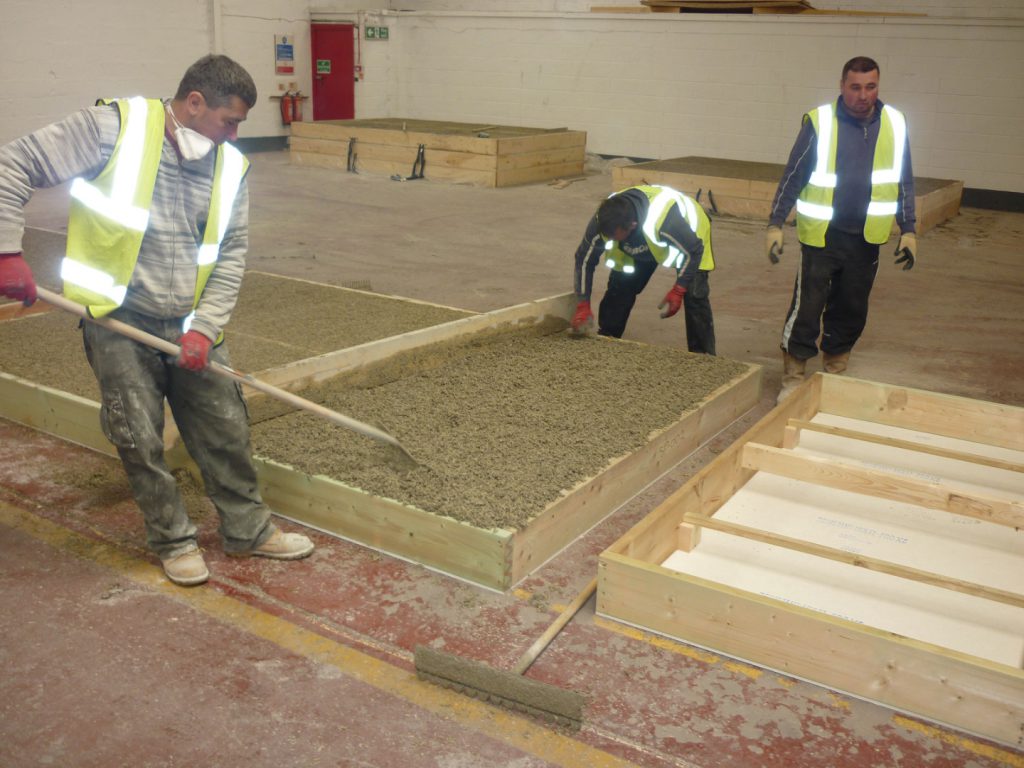
To reduce any potential dust emanating from the uncoated hempcrete panels and for fire protection, the inner face of each Hemcrete® panel was lined with a thin vapour-permeable unpainted magnesium silicate board (Multi-Pro)[3], even though it was likely that this would reduce the buffering capability of the hemp-lime. The outer face of the building was covered with a vapour permeable membrane, wood fibreboard and rodent-proof wire mesh with an added layer of Multi-Pro board against collision damage at floor level.
Access to each of the larger object storage rooms is through both steel roller shutter doors and personnel doors; each storage room also has a fire exit. Free-standing stair access, balconies and railings were installed front and back and an object-only lift installed at one front right corner. Reach truck access is through gates in the safety railings.
Control of the internal environment
https://dx.doi.org/10.15180/150405/011It was always envisioned that some active mechanical system would need to be included in the environmental control of the store. This was intended to provide very energy-efficient management of the ambient environment in conjunction with the passive regulation by the building fabric. Ventilation to outside was considered necessary as the RH levels within the hangar would always be higher than those inside the repository; moisture stored in the hemp-lime walls would need to be dried out periodically. A Trend® IQ3 building management system (BMS) and two Airflow® air-handling units (AHU) were installed to circulate external cool dry night-time or warm daytime air depending on humidity requirements within the store. Services were run externally with only vents and monitors inside each room.
It was also of great importance to have a very accurate monitoring system as a source of data to research the effectiveness of the store, and to monitor the day-to-day conditions for maintaining the care of the objects. In fact, two monitoring systems were included: a Carnego® monitoring system for the BMS (14 combined T and RH sensors – two in each storeroom, one in the hangar and one external) was installed and managed remotely by the design engineers. Combined RH and T sensors were also installed as part of the museum’s bespoke environmental monitoring system[4], managed by Conservation staff.
Teething problems
https://dx.doi.org/10.15180/150405/012Initial issues with moisture levels were detected by the more sensitive Hanwell® sensors. Both external BMS T and RH sensors were faulty as was the control software running the AHU fans, resulting in warm moist daytime air being drawn into the store rooms instead of cool dry air at night. There was unanticipated moisture from both the drying concrete floor mass, affecting mainly the ground floor store, and the hemp-lime panels. By July 2012, less than three months before the first objects were to be relocated to the store, RH levels were close to 80 per cent on the ground floor, 70 per cent on the first floor and 65 per cent on the top floor – measurements which fell outside the museums’ own acceptable range.
These teething problems required the museum to employ unexpected active mechanisms to reduce the RH levels at least in the short term. Portable dehumidifiers and air conditioners were deployed along with a slight elevation of the ambient temperature. After sensors were replaced, software updated and heaters rewired, operation of the AHU system assisted in reducing the RH to between 40–50 per cent on all floors (Jankovic, 2013). Fluctuations in RH were also considerably reduced through the replacement of faulty dampers, which had caused an imbalance in the airflow from the ductwork. However, both fan and heater operations continued to cause fluctuations between 5–10 per cent/24 hours. RH levels also rose when the dehumidifier condensate tanks went unemptied[5]; this was resolved by connecting the equipment to the hangar’s drainage channel to achieve continuous draining.
With the seasonal rise of temperature, the heaters were turned off in May 2013 which resulted in a slow rise of RH to 60 per cent in the ground floor store room. In order to decrease RH fluctuations, it was decided to allow the building to drift passively through the spring and summer seasons so the entire air handling system was switched off. The store remained in passive mode until March 2014, when the RH levels, which had drifted slowly upwards at a rate of around one per cent per month, rose above seventy per cent on the ground floor. This was considered to be too high and an attempt was made to reinstate the HVAC system with minimal success as handover and training to run the system had never occurred.
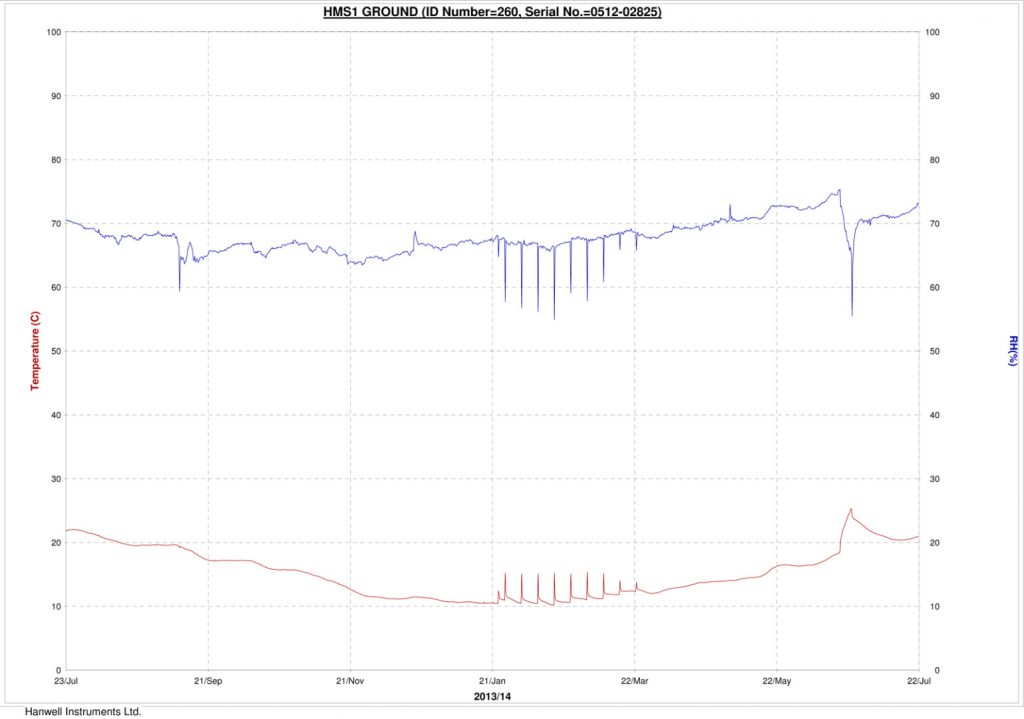
Initially, from August 2014, the RH was again reduced using portable dehumidifiers and air conditioners. The original designer was contracted to reinstate the air handling and heating system and he also provided training to the site’s Estates team. Full running of the system was reinstated five months later although mild and damp external conditions meant that initially four hours of daily heating was primarily used to reduce the RH levels. This resulted in daily rapid fluctuations of 10–15 per cent. Fluctuations were reduced once drier external air could be utilised along with heating. Levels were stabilised over three months on the ground floor to 60 per cent and 55–60 per cent on the other two floors. In April 2015, RH regulation was returned to the passive mode and fluctuations were virtually eliminated. Again the RH rose very slowly until, by July 2015, it was around 68 per cent on the ground floor. Because the external air was rarely drier outside the hangar than that within the store due to the wet summer, a single dehumidifier with humidistat set to sixty per cent, connected to the hangar’s drainage, was installed in the ground floor store room. This resulted in stabilisation of RH to just above sixty per cent with virtually no fluctuation whatsoever. RH levels on the other two floors have also dropped slightly, indicating moisture movement through the interconnected porosity of the low-density hemp-lime walls.
Discussion
https://dx.doi.org/10.15180/150405/013To date, the hemp-lime walls have been shown to be very effective moisture buffers. Even when the mechanical system was malfunctioning, introducing moisture-laden air into the store rooms instead of dry air, the interior RH levels never rose close to the extent of the environmental conditions in the hangar. Once stabilised at an acceptable RH level and with the mechanical system switched off, the building has demonstrated consistently excellent RH stability.
But as the hemp-lime functions as a buffer not as a controller of RH, some method of moisture removal from the hempcrete continues to be required in order to bring the RH back down to preferred values. Monitoring data from D2 hangar shows RH levels outside the store to be generally between 70–100 per cent[6]. As the RH inside the store is lower than that in the hangar, moisture cannot be transported out through the hempcrete walls and into the hangar; the vapour-permeable membrane fitted between the hempcrete walls and the external wood fibreboard also prevents moisture movement from the hangar back into the hempcrete material. In order to remove moisture, the air handling plant runs cycles of heating and air flow, introducing drier air into the storerooms and extracting damp air. This does gradually reduce RH levels but creates continuous fluctuations which could contribute to the physical deterioration of organic materials over time, although the fluctuations are of short duration thanks to the buffering effect of the hygroscopic materials.
As collections are now stored in the rooms, the RH must be controlled within the group’s set parameters and should not be allowed to rise above seventy per cent for any extended period of time. So far, the air handling system has only reduced the RH to 55–60 per cent during the winter months, with the RH then rising to the maximum level over six to eight months of passive operation. If the moisture removal process is followed as originally intended, cycling dry air and heat through the winter season to reduce the RH, then allowing a passive drift for the remainder of the year, this should provide an acceptable level of control. However, at present the air handling cycling must be run by an operator who monitors external conditions; the building management system software is not sophisticated enough to check or predict drier external conditions in order to turn on the air handling units. As drier conditions generally occur at night outside normal working hours, this means the system does not fully run as designed. Using the heating to reduce RH rather than external dry air has been shown by the monitoring to cause greater fluctuations in RH. Because of the successful (lower RH level and fewer fluctuations) trial with the current dehumidification equipment, discussion is underway regarding the possible installation of dehumidification units in the ducting. These could be operated during working hours, drying external air before introducing it into the storerooms, mimicking the original concept but adding a little more engineering.
When the mechanical system has been running, its operation has been very low-cost. Currently it runs with electrical consumption at 20,397.5kWh against a predicted 24,320kWh, which is less than a third of the running costs and emissions of a conventional environmentally controlled museum store of the same size (Moore, 2013). When in passive mode, the running costs are negligible; costs of running the dehumidifier have yet to be calculated but it will not consume even close to the same power as the fully operating system.
The hempcrete museum store took a longer time to dry out than anticipated by either the contractors or the museum project team. But, because the hempcrete has demonstrated such excellent hygrothermal characteristics, objects stored in the building were not put at risk during the stabilisation period, as shown by the monitoring data. RH fluctuations have stayed within established parameters for the materials stored; RH levels have been slightly high but never in the range to support mould growth or promote corrosion.
Continued monitoring of the hempcrete store may show that, as the building materials continue to cure over time, RH levels do not rise quite as high so that operation of the air handling system will be required less frequently. Or the hemp-lime panels may now be ‘pre-conditioned’, with a memory of an RH level of 55–70 per cent and it may never be possible to prevent the eventual drift back up to 70 per cent. However, operating the building as is done presently still has given the Science Museum Group a very effective and efficient environmentally controlled store at a minimal running cost and an excellent prototype for future storage building projects.
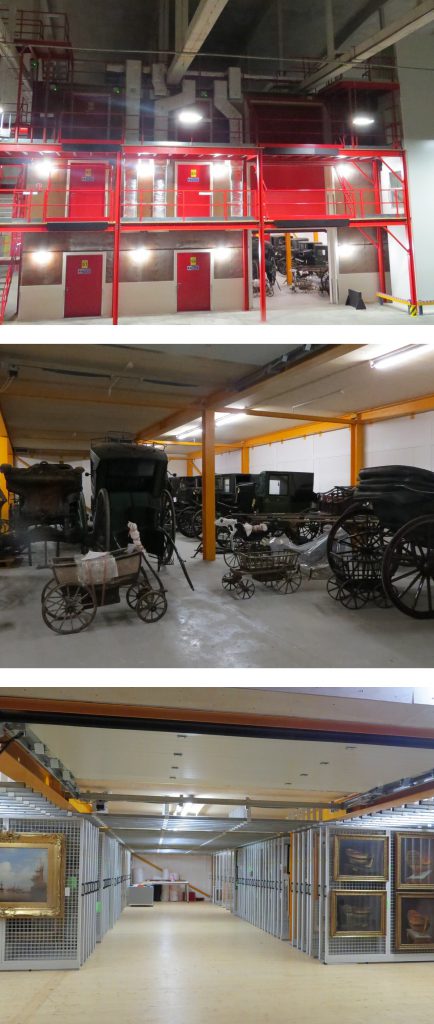
Conclusions
https://dx.doi.org/10.15180/150405/014The Hempcrete Museum Store is a step forward in providing sustainable controlled environment museum storage. While construction costs were approximately ten per cent higher than a traditionally constructed museum store (Moore, 2013), its running costs are less than half of a climate controlled space of the same size. The bio-based materials used have reduced the carbon footprint of its construction and the hemp-lime will continue to lock up CO2 for the duration of its use. The environmental conditions within the store are stable; when the building is in passive mode there are virtually no fluctuations in RH or temperature. When the mechanical air handling system is running, the buffering ability of the hygroscopic building material reduces the extent of fluctuations caused by heating or fan operation.
The store won three major awards in 2013 for innovation and sustainability both in the museum and the building industry sectors: Greenbuild Awards, Best Workplace New Build; Museums and Heritage Awards, Sustainability; Chartered Institute of Building (CIOB) South West Built Environment Awards, Innovation[7].
The Hempcrete Museum Store may be used as a prototype for developing further sustainable museum storage for the Science Museum Group. Wroughton needs new large storage buildings to replace the ageing hangars. Building storehouses with hygroscopic materials may be part of the solution since they naturally provide the stable environment that collections require to ensure long-term preservation. Areas for further research include looking at passive alternatives to mechanical and engineering systems to reduce moisture storage and investigating more porous materials to coat interior walls. The possibility of fine particulate matter[8] drifting out of unsealed hemp-lime also needs to be established as well as the potential effect that the alkaline nature of hempcrete might have on acidic pollution from degrading paper, plastic and wood. An internal environment in a detached building may react differently to that of this store built inside a hangar, or alternatively building design may need to mimic the ‘building within a building’ concept. Some of these factors may be answered through continuing research being undertaken by the author at the University of Bath. Others may only be answered by building another storage building.
Acknowledgments
I would like to thank Louisa Burden, Matt Moore and Dennis Kelles-Krause, Science Museum Group, for their assistance in writing this article. I also wish to thank my supervisors, Dr Michael Lawrence and Dr Stephen Lo, University of Bath, Department of Architecture and Civil Engineering for their support and encouragement.
Appendix A
https://dx.doi.org/10.15180/150405/015The Hempcrete Museum Store project design team:
External Contractors
Ian Pritchett, Technical Director and Vice Chairman, Lime Technology
Piers Ashley-Carter, Project Manager, Hemcrete Projects Ltd
Dr Lubo Jankovic, Director, Emission Zero R&D
Jeremy Collins, Structural Engineer, Sylva Group
Andy Aram, Quantity Surveyor
Dr Mike Lawrence, Consultant, BRE Centre for Innovative Construction Materials
Simon Kirton, Director, Emission Zero A&E
Internal SMG Project Management team
John Bevin, Director of Estates (retired)
Louisa Burden, Head of Conservation and Collections Care
Matt Moore, Head of Sustainable Development
Tim Procter, Curator of Archives, National Railway Museum
Marta Leskard, Conservation and Collections Care Manager
Darren Hopkins, Estates Manager
Simon Chambers, Project Manager
Appendix B: Manufacturers and suppliers
https://dx.doi.org/10.15180/150405/016Software
Air handling and monitoring equipment
- Hemclad® Limetec group
- Tradical® Hemcrete® Lhoist Group, Tradical® HB- lime binder, Tradical® HF- hemp
Air handling and monitoring equipment
Tags
Footnotes
Back to text
Back to text
Back to text
Back to text
Back to text
Back to text
Back to text